Watch the video and download technician notes from the Education in Chemistry website: rsc.li/EiC618ec
We take a look at some of the unexpected insights that changes of state can provide us with. It’s a lot more interesting than watching paint dry.
Kit
- Data logger with temperature probe, capable of live graphing, resolution ±0.1°C and sample frequency at least once per second
- Test tube
- 500 cm3 beaker
- Ice (about 200 g)
- Salt (about 25 g)
- Tap water (about 200 cm3)
- Deionised water (about 5 cm3)
- Retort stand and clamp
- Stirring rod or magnetic stirrer
Preparation
Make a water bath by placing the tap water in the 500 cm3 beaker – if a stirrer is available then you can place the beaker on it and add a magnetic follower. Use the clamp and retort stand to hold a test tube containing 5 cm3 of deionised water above the the water bath. Set up the data logger to record for at least 1200 seconds (20 mins) (eg 2 samples per second recording 2400 samples). Connect and place the temperature probe in the test tube.
Measure out about 5 teaspoons of salt (20–25 g) and 200 g of ice.
In front of the class
Project the temperature–time graph from the data logger and add the salt and half the ice to the water bath. Ask students to predict the temperature of the bath and to suggest what will happen to the temperature of the water in the test tube once submerged. Mini-whiteboards are a great tool for establishing preconceptions at this point.
Stir the water bath throughout the experiment and start data collection. Lower the test tube and probe into the bath so that the water within is submerged. The temperature on the screen will begin to drop. Some students will have predicted that the temperature will drop continuously through zero and are likely to feel vindicated as the water supercools. You can draw their attention to the fact that the water is still liquid by gently moving the probe.
This sets up a really satisfying twist for the class. The water may continue to supercool for up to three more minutes. Then, a shocking temperature spike appears as an ice crystal nucleates and rapid freezing occurs exothermically. The temperature then locks onto 0°C until the freezing has completed, and only then resumes decreasing towards the temperature of the water bath, which may by now require more ice.
After about ten minutes, lift the tube out and replace the contents of the bath with lukewarm tap water. Lower the test tube back in, but do not disturb the probe, which should remain frozen within the ice. The temperature will rise, lock onto 0°C until the thaw is complete and then continue towards the ambient temperature.
By zooming in on the graph, the random error in the probe’s readings will be evident – these can be reduced by taking an average of the readings in the straight-line section. The average values for freezing and melting will agree within the uncertainty of the probe.
Teaching goal
This simple demonstration summarises many concepts that underpin the whole of chemistry.
For the kinetics, we see that the average speed of the particles’ motion – which is proportional to the temperature of the substance – has dropped to the point that the attraction between them wins out over the motion that shakes them apart. The liquid solidifies.
For thermodynamics, we see that bond formation (hydrogen bonds, in this case) is exothermic. During the process of freezing, heat energy from bond formation is released at the same rate it is lost to the cooler surroundings. The sodium acetate ‘hot ice’ demonstration is a useful example to support students who struggle with the concept of heat being released from something that’s ‘cold’.
For thermodynamics, we see that bond formation (hydrogen bonds, in this case) is exothermic. During the process of freezing, heat energy from bond formation is released at the same rate it is lost to the cooler surroundings. The sodium acetate ‘hot ice’ demonstration (rsc.li/2yEkpmy) is a useful example to support students who struggle with the concept of heat being released from something that’s ‘cold’.
In the delayed freezing, we see the study of chemistry relies on an understanding of the interplay of kinetic and thermodynamic effects – just because something should happen, doesn’t tell us anything about how fast it happens. In this case, for an ice crystal to form, an energy barrier has to be overcome. This barrier reflects loss of positional entropy during the formation of a relatively-ordered cluster, the breaking of hydrogen bonds in the liquid and reorientation into the cluster, and work done against surface tension of the cluster as it grows.
Finally – as students can often misperceive the models they learn as ‘facts’ that turn out to be ‘lies’ – it’s worth pointing out that while we can describe simple models to visualise this process, our understanding of it is still developing. And our ability to make quantitative models match the data is always improving.
Downloads
Freezing water: technician notes
Word, Size 52.59 kbFreezing water: technician notes
PDF, Size 45.69 kb
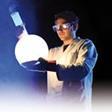
No comments yet