The same chemistry that drives the famed cobalt traffic light and the pink catalyst reaction also drives another colour-changing reaction – the blue and gold reaction. This reaction is an excellent introduction to reversible reactions because of the repeated rapid switches between shades of blue, green, yellow and red, with accompanying fizzing and precipitation.
Get started
Watch a demonstration of this experiment and download the technician notes from the Education in Chemistry website: rsc.li/45wFtb2
Scientists exploring the famed cobalt traffic light (rsc.li/3S5OK77) or pink catalyst reaction discovered that the same chemistry could drive other colour changes, and the blue and gold reaction was born. This reaction is an excellent introduction to reversible reactions because of the repeated rapid switches between shades of blue, green, yellow and red, with accompanying fizzing and precipitation.
Kit
- 30 cm3 of 1 M potassium sodium tartrate solution
- 100 cm3 of 3% (10 vol) hydrogen peroxide solution
- 1 cm3 of 0.5 M copper(II) sulfate solution
- Kettle and large beaker (eg 1 L) to make a water bath
- Magnetic stirrer and stirrer bar
- 250 cm3 beaker (preferably tall form) for the reaction
- Boiling tube for heating an initial portion of the hydrogen peroxide solution
- Thermometer (with a range of 0–100°C)
- White tile
Preparation
Make up 100 cm3 of fresh 10 vol hydrogen peroxide (10 vol solutions do not keep well because the decomposition inhibitor is less effective when diluted).
Place 30 cm3 of 1 M potassium sodium tartrate in a 250 cm3 beaker (to make this fresh from the tetrahydrate salt dissolve 8.46 g of solid in 30 cm3 of water). Add 15 cm3 of 10 vol hydrogen peroxide to a boiling tube and place both the beaker and the boiling tube in a 1 L beaker. Keep the remaining hydrogen peroxide ready at room temperature. Add a thermometer to the tartrate solution and add hot water from a kettle to the 1 L beaker to bring the solutions to 50–60°C in time for the experiment.
Preparation
Make up 100 cm3 of fresh 10 vol hydrogen peroxide (10 vol solutions do not keep well because the decomposition inhibitor is less effective when diluted).
Place 30 cm3 of 1 M potassium sodium tartrate in a 250 cm3 beaker (to make this fresh from the tetrahydrate salt dissolve 8.46 g of solid in 30 cm3 of water). Add 15 cm3 of 10 vol hydrogen peroxide to a boiling tube and place both the beaker and the boiling tube in a 1 L beaker. Keep the remaining hydrogen peroxide ready at room temperature. Add a thermometer to the tartrate solution and add hot water from a kettle to the 1 L beaker to bring the solutions to 50–60°C in time for the experiment.
Safety and disposal
- Wear eye protection.
- Hydrogen peroxide can cause serious eye damage. CLEAPSS members should see RB045 before diluting 100 vol (30%) hydrogen peroxide.
- Copper (II) sulfate is harmful if swallowed and can cause serious eye damage. Avoid contact with skin.
- Dilute all solutions with plenty of water and dispose of all diluted solutions in a foul-water drain.
Safety and disposal
- Wear eye protection.
- Hydrogen peroxide can cause serious eye damage. CLEAPSS members should see RB045 (bit.ly/46MzwYE) before diluting 100 vol (30%) hydrogen peroxide.
- Copper (II) sulfate is harmful if swallowed and can cause serious eye damage. Avoid contact with skin.
- Dilute all solutions with plenty of water and dispose of all diluted solutions in a foul-water drain.
In front of the class
Wear eye protection. Place a white tile on a magnetic stirrer, place the stirrer bar in the 250 cm3 beaker of potassium sodium tartrate solution, add the 15 cm3 of heated hydrogen peroxide solution from the boiling tube and begin stirring. Monitor the temperature. The reaction is initiated by the addition of approx 1 cm3 of 0.5 M copper(II) sulfate. This is best done when the solution reaches 50°C.
The colour of the copper(II) sulfate would be expected to be near-colourless when diluted to this extent by adding into the tartrate/peroxide mixture. Instead a deep blue colour is observed as copper(II) tartrate complex is formed. Lots of bubbles of oxygen and carbon dioxide are released and after ≈10 seconds the solution changes from blue to an opaque, golden-orange-yellow coloured precipitate. The temperature will rise rapidly at this point to ≈65°C. On addition of 10 cm3 of room temperature 10 vol hydrogen peroxide, the blue colour will return before the reaction repeats and cycles back to the orange precipitate. Add further aliquots of hydrogen peroxide to cycle the reaction until the tartrate, or your audience, becomes exhausted!
The reaction takes longer to repeat if you allow the temperature to drop, but this can be compensated for by adding the peroxide quickly enough after the precipitate finishes forming to maintain the temperature at 60–70°C throughout the experiment. Avoid allowing the temperature to rise too much because this inhibits the cycling of the reaction.
Tips
- Work against a white background. A tall form beaker, if you have it, will show the colours at their best from the smallest volume of solution at the start.
- Maintain a temperature of 60–70°C by using the exotherm that accompanies production of the precipitate. If you stray from this range the latency time will increase.
- The demonstration can be scaled up or down but the temperature drops faster when the mass of solution is smaller, and the volume of the Cu(II) solution becomes difficult to measure accurately.
- Adding more Cu(II) (eg 2 ml of 1 M) dramatically reduces latency time to almost zero, and makes a heavier precipitate. This will mean that the blue will be more intense, but the orange can appear muddier in colour.
- Higher tartrate concentrations reduce the latency time, but higher peroxide concentrations increase it. So, by starting with 30 cm3 of peroxide followed by 20 cm3 additions, the first latency time should be ≈20 seconds.
Teaching goal
When executed well, the cobalt variation of this reaction demonstrates catalytic effects superbly, with differently coloured complexes formed during the reaction before the original colour returns. However, this copper variation consumes only a small proportion of the tartrate ions each time, so it makes for a visually appealing demonstration of the reversibility of reactions and demonstrates aspects of transition metal chemistry (changes of colour, variable oxidation states, complex formation and catalytic behaviour).
On addition of the copper, the solution goes from near-colourless to blue as intensely coloured copper–tartrate complex ion forms (Equation 1). After a short latency period, the copper acts as a catalyst (Equation 2) for the decomposition of peroxide (the two can react without the presence of tartrate), and in the oxidation of tartrate
(Equation 3). Eventually, the ratio of [tartrate]:[H2O2] becomes high enough and the Cu(II) complex becomes a reactant and is reduced to the orange precipitate. The orange precipitate looks like the copper(I) oxide formed in a positive Benedict’s test but is more likely to be a copper(I)–peroxo–tartrate complex of Cu2O.
When more peroxide is added the ratio flips back again and reoxidises the Cu(I) to Cu(II), which causes the chain of reactions to start over, this time from a slightly higher pH. The reason the reaction tends to slow over time would seem on first glance to be explained by the reduction in tartrate concentration, but higher temperatures and higher pH also inhibit formation of the precipitate (one reason it’s not recommended to go too much higher than 70°C).
Teaching goal
When executed well, the cobalt variation of this reaction demonstrates catalytic effects superbly, with differently coloured complexes formed during the reaction before the original colour returns. However, this copper variation consumes only a small proportion of the tartrate ions each time, so it makes for a visually appealing demonstration of the reversibility of reactions and demonstrates aspects of transition metal chemistry (changes of colour, variable oxidation states, complex formation and catalytic behaviour).
On addition of the copper, the solution goes from near-colourless to blue as intensely coloured copper–tartrate complex ion forms. After a short latency period, the copper acts as a catalyst for the decomposition of peroxide (the two can react without the presence of tartrate), and in the oxidation of tartrate. Eventually, the ratio of [tartrate]:[H2O2] becomes high enough and the Cu(II) complex becomes a reactant and is reduced to the orange precipitate. The orange precipitate looks like the copper(I) oxide formed in a positive Benedict’s test but is more likely to be a copper(I)–peroxo–tartrate complex of Cu2O.
When more peroxide is added the ratio flips back again and reoxidises the Cu(I) to Cu(II), which causes the chain of reactions to start over, this time from a slightly higher pH. The reason the reaction tends to slow over time would seem on first glance to be explained by the reduction in tartrate concentration, but higher temperatures and higher pH also inhibit formation of the precipitate (one reason it’s not recommended to go too much higher than 70°C).
More resources
- Discuss intermediates and catalysis with 14–16 or post-16 learners using the cobalt variation of this demonstration.
- Embed transition metal properties, such as chelation, complexes and more, in your 16–18 students with a luminescent copper catalysed practical.
- Develop 14–18 learners’ understanding of heterogeneous catalysis using this copper catalysed experiment.
Downloads
Out of the blue technician notes
Editable handout | Word, Size 0.45 mbOut of the blue technician notes
Handout | PDF, Size 0.15 mb
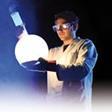
2 readers' comments