Mould your students’ understanding of polymers with these misconception busters and wealth of teaching ideas
Polymers form materials that can be bent and shaped like metals, but are lighter, more durable, cheaper and generally less reactive. They can be designed to be electrical insulators or conductors. They can also be anything from waterproof to brittle, pliable, coloured, opaque, transparent, sticky or non-stick. Just name the property and it’s probably been developed. All plastics are polymers, and man-made polymers are termed synthetic.
What you need to know
Polymers provide an ideal context to discuss and consolidate ideas about structure, bonding and properties. It can be difficult for students to understand that intramolecular (covalent) bonding and intermolecular forces are both caused by electrostatic attraction. Intermolecular forces, such as van der Waals, hydrogen bonding and ion–dipole interactions, play an important part in the structure and function of polymers, both synthetic and natural.
Revisit the reactivity of small, unsaturated hydrocarbons using ethene as a prototype. Use molecular models to illustrate the double bond opening of addition reactions. Then extend this to make a class ethene addition polymer – poly(ethene). The monomer-polymer card game nicely reinforces the link between the monomer and the addition-polymer name.
Revisit the reactivity of small, unsaturated hydrocarbons using ethene as a prototype. Use molecular models to illustrate the double bond opening of addition reactions. Then extend this to make a class ethene addition polymer – poly(ethene). The monomer-polymer card game (rsc.li/3M09EkY) nicely reinforces the link between the monomer and the addition-polymer name.
Use modelling to help your students understand the concept of adding together monomer units to form polymers. You can model polymers with paperclips, using just one colour to show a homopolymer forming, or two colours to show a heteropolymer. Ask your students what happens to the density and strength of the resultant materials if the linear chains start to branch off? Have them research different forms of poly(ethene), which is used to make materials from plastic bags to industrial strength piping.
Synthetic polymers are broadly divided into two categories based on how they respond to heating. Thermoplastics can be reshaped when heated due to the relatively weak intermolecular forces between the polymer chains. By contrast, thermosetting plastics have strong cross-links between the polymer chains and retain their shape when heated.
Suggestions for teaching
- Polymers are materials made from long-chained molecules, formed from the reaction of many smaller monomer molecules.
- Changes in the structure of synthetic polymers, from linear to branched chain poly(ethene), for example, can lead to changes in properties such as density and strength.
- Biological polymers, such as proteins and DNA, are formed by reversible condensation polymerisation. Different intermolecular forces are essential in the structure and function of biological systems. For example, hydrogen bonding is strong enough to dictate secondary structure, but weak enough to allow biological function, such as in DNA replication.
- Small changes in natural protein structure can lead to diseases.
Use the formation of condensation polymers like esters for a good problem-solving exercise. What forms if we replace the single carboxylic acid molecule with a diacid? Allow students to use non-permanent whiteboard markers to make sketches. I find students have less fear of making errors when sketching out structures this way. Then take the next step and replace the monoalcohol with a dialcohol. They should then see the possibilities of polyesters by themselves. This condensation reaction is similar enough to extend to amide links and proteins.
Adding links between the chains can strengthen polymer materials. The gloopy polymer based on the sodium alginate suspension in Gaviscon® is made solid by adding divalent metal ions, bolstering the weak intermolecular attractions. Adding simple substances can also disrupt polymer networks. Baby oil will cause filled balloons to burst as the polymer chains are pulled apart. Acetone will soften expanded polystyrene, allowing the trapped air to escape and vast volumes of the packing material to ‘disappear’ into a beaker.
While synthetic polymers are usually relatively simple homo- or co-polymers (one or two monomer units), proteins can also be formed from reversible condensation reactions with many monomers. Think of the gain-of-function that enzymes and proteins have from using 20 different monomers. Proteins are fascinating from the perspective of intermolecular forces and structure. Secondary structures – the 3D structural elements – are formed because of the primary structures – the linear chain of amino acids. The relatively weak intermolecular forces can be disrupted by pH changes and temperature which leads to denaturing. Strong disulfide bridges join some protein chains to form a sulfur−sulfur covalent bond. Disrupted secondary structures can lead to misfolded proteins, or protein aggregation. This malformation can lead to diseases such as Alzheimer’s.
DNA is simpler to examine in terms of intermolecular forces. Each polymer chain has a sugar−phosphate repeating unit with bases providing the hydrogen bonded cross links to form the famous double helix. The DNA helix can be unzipped by molecular machines in the cell, or by heating up to 98°C in the polymerase chain reaction.
Clearing up misconceptions
Be careful with your use of language around the terms bonds and forces. In general, limit your use of the term bonds for intramolecular covalent interactions and the term force for intermolecular interactions (eg van der Waals). Natural proteins can be used to form glues – think of denatured casein from milk and collagen (used to connect our skeleton together), which illustrates the potential strength of these forces holding a protein’s secondary structure. It would be worthwhile discussing why proteins can form discrete molecular structures with your students.
Hydrogen bonding is a particularly strong intermolecular force, sometimes with the implication that there is something special or different. However, hydrogen bonding is electrostatic in nature, a fact worth emphasising.
The ability to biodegrade depends on several factors. Condensation polymers can be hydrolysed. Addition polymers, like poly(ethene), can biodegrade if the chains have a molecular weight of less than 500.
Resources for your classroom
- Show your students how two liquid monomers come together to form a visible fibre with the formation of nylon. You can also use this resource to highlight the (weaker) intermolecular forces in polystyrene by breaking down the structure using acetone.
- Teach your learners to make plastic from natural material potato starch.
- Demonstrate the stickiness of the intermolecular forces in proteins by denaturing milk proteins to make glue.
- Test your learners’ understanding of how addition polymers are structured and named with this classroom activity, poster and fact sheet.
Resources for your classroom
- Show your students how two liquid monomers come together to form a visible fibre with the formation of nylon (rsc.li/3M09EkY). You can also use this resource to highlight the (weaker) intermolecular forces in polystyrene by breaking down the structure using acetone.
- Teach your learners to make plastic from natural material potato starch: rsc.li/3M0k7g9
- Demonstrate the stickiness of the intermolecular forces in proteins by denaturing milk proteins to make glue: rsc.li/430jBF8
Duncan Short has taught chemistry and science for a number of years in Fife and currently teaches in Falkirk
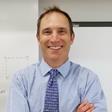
No comments yet