Discover the chemistry needed to power our future
‘If you love solving challenging problems, then battery development is a great place for you,’ says Serena Cussen, head of the University of Sheffield’s department of materials science and engineering. ‘To me, it’s the richest playground in the world. I get to delve into so many aspects of science and engineering that I love.’
Today’s electric vehicles (EVs) are powered by lithium-ion batteries, which shuttle lithium ions through an electrolyte between the negative anode and positive cathode, with the direction of travel changing during charging. As the number of EVs on the road continues to increase at breakneck speed, so too does the need for batteries that can not only charge more quickly but also keep their charge for longer. Improving batteries while maintaining their safety, affordability and high performance is no mean feat.
The race is on to find ways to reduce or eliminate reliance on cobalt in EV batteries
Anodes in lithium-ion EV batteries are generally graphite materials while cathodes tend to be made from layered transition metal oxides containing nickel–manganese–cobalt (NMC) or nickel–cobalt–aluminium (NCA). Most common are NMC cathodes, but NCA batteries are used in some higher-end cars. Meanwhile, lithium cobalt oxide (LCO) cathodes, used in the first commercial lithium-ion batteries over 30 years ago, can also be used for smaller EVs but are mainly used for portable electronic devices, such as smartphones and tablets.
In your class
Give context for electrochemical cells by highlighting their applications in everyday technology. Provide the lithium-ion cell as an example and reinforce your learners’ understanding of rechargeable batteries when revising oxidation and reduction. The accompanying resource download also tasks learners with calculating the electromotive force (EMF) of the cell during discharge and consolidating their understanding of terminology and processes used in electrochemistry, making distinctions between galvanic and electrolytic cells.
Next generation cathodes
The race is on to find ways to reduce or eliminate reliance on cobalt in EV batteries. The metal is prohibitively expensive due to limited availability and it comes with serious ethical concerns. The Democratic Republic of Congo provides over 60% of the world’s cobalt, and political instability there has led to mining operations using child and slave labour and causing environmental damage.
Serena is the principal investigator of a project that aims to develop cobalt-free battery materials as well as developing cathodes that will increase durability, range and acceleration for the EV market. The project’s academic researchers work with industry partners to make sure that proposed technology can directly transfer to commercial processes. ‘We’re trying to make sure the materials we discover today will go into devices tomorrow,’ she says.
The project has been developing nickel-rich cathodes, including a lithium nickel oxide (LNO), which is nearest to market. The team are now looking at large-scale manufacturing and testing of the cathode material. Increasing the nickel content makes the cathodes more complex and also brings in structural and thermal instabilities. Preventing the degradation processes that occur in nickel-rich layered oxides is crucial for them to realise their full potential as high-energy density cathodes.
The price of nickel has rocketed in recent months. ‘Our reliance on these kinds of elements is becoming more and more precarious, so we have to develop new cathodes that could potentially replace them,’ explains Serena. So, the team is looking into a new class of manganese-rich cathodes called disordered rock salts, which need neither nickel nor cobalt and are ‘very promising in terms of delivering high capacities’. But there are challenges with stability and long-term performance. ‘We are learning more about that by using advanced methods of X-ray diffraction and total scattering to understand at a fundamental, atomic level how materials behave as you cycle them,’ Serena says.
The problem of degradation
Clare Grey from the University of Cambridge runs a degradation project, looking into lithium-ion battery cells containing high nickel-content NMC and graphite. ‘We’re trying to understand what the modes of degradation are. The particularly challenging thing is you can have degradation on the cathode, but the degradation products affect the anode, so you have to study the whole cell,’ she explains.
Nickel in an unstable oxidation state leads to a whole series of reactions. For example, reactive singlet oxygen ‘eats up’ the electrolyte to produce protons which then damage the protective coating on the anode, which, in turn, consumes more lithium ions.
The project uses a variety of techniques to study all the processes, including electron microscopy to look at surfaces and NMR spectroscopy to investigate what goes on inside the electrolyte. Using in situ NMR, the researchers can track lithium ions in real time. Another ‘nice experiment’ is to shine a light at a battery and do optical scattering. ‘We can watch the lithium ions going in and out of particles,’ says Clare.
Download this
Worksheet, for age range 16–18
Use this resource to review electrochemistry and apply understanding to lithium-ion cells.
Download the student worksheet, teacher notes and answers from the Education in Chemistry website: rsc.li/3utr2o8
Lithium metal oxide batteries have reached maximum capacity when it comes to lithium shuttling, explains Clare. ‘We can almost pull all the lithium out and almost put it all into the graphite with very little irreversibility. If we really want to make batteries that have an impact, we have to make them last longer. Some of the challenges are with processes specific to particular chemistries and some are general. Each generation tends to bring along a new series of problems, and these nickel-rich materials with less cobalt are more prone to losing oxygen and electrolyte degradation.’
Clare’s team is working on sodium-ion batteries as a more sustainable and cheaper alternative to lithium. Where the anode on a lithium-ion battery uses a copper current collector, the team have found they can use aluminium instead for sodium batteries. ‘We’re trying to think about materials that are inherently more sustainable from the beginning,’ she says.
Supercharged
In 2019, Clare cofounded a University of Cambridge spin-out, Nyobolt, to develop fast-charging batteries using a niobium tungsten oxide (NWO) cathode material. The proprietary NWO chemistry was developed in Clare’s research group. The aim is to develop batteries for electronics and robotics, as well as for heavy industry.
Investigate batteries further with the 2022 global experiment
Perfect for your younger students, Take charge: a global battery experiment gives them the opportunity to build and test their own coin batteries, as well as exploring how they can help build a more sustainable future. It’s so easy, get started today.
Meanwhile, another University of Cambridge spin-out called Echion Technologies has created new active anode materials containing niobium. Its mixed niobium oxide (XNO) material promises ‘safer, faster charging and longer-lasting batteries,’ says Alex Groombridge, chief technology officer and cofounder. XNO’s structure allows reversible and ‘superfast’ lithium-ion diffusion within microcrystals.
‘We’re looking at batteries that can last for more than 10,000 charge and discharge cycles, whereas a standard battery that you might find in your phone will only last for a few hundred before it starts to degrade,’ Alex says. The technology is primarily aimed at industrial and commercial battery applications, such as electrifying diesel trains or powering ‘monstrous’ mining vehicles.
With so many aspects to focus on and the chance to make a difference, many students want to get into battery research
Graphite anodes can cause severe safety problems when you try to fast-charge a battery, Alex explains. ‘When graphite takes on lithium ions, the voltage – the chemical potential at which this happens – is close to when lithium ions become lithium metal. So, if you try to do it very fast, your lithium ions have more of a tendency to become lithium metal.’ This metal can cause a short circuit between the anode and the cathode or enhance degradation. ‘Standard lithium-ion cells simply can’t fast-charge,’ he says. ‘When you fast-charge with our materials it’s impossible to make lithium metal deposits, so the chemistry itself is safer.’ The company has gone from making small amounts of its niobium anode material in a lab in 2017 to now building a new factory for several thousand tonnes per year.
Across the Atlantic, US firm EC Power has collaborated with Pennsylvania (Penn) State University researchers to adapt existing lithium-ion batteries to make them fast-charging. Their technology allows them to charge a high-energy density EV battery to 70%, equivalent to a range of more than 300 kilometres, in just 11 minutes. The team is now working to demonstrate charging of batteries from empty to 80% in 5 minutes says Chao-Yang Wang from Penn State.
The team uses stacks of anodes and cathodes interspersed with ultra-thin layers of foil. It uses temperature modulation to rapidly heat the battery cell and takes advantage of thermally enhanced electrochemical and transport processes to carry out fast-charging. The technology can also work in different types of batteries, including solid state ones.
Fast-charging batteries can be smaller so more EVs could be made with the same quantity of materials. ‘The most effective path to battery sustainability is to reduce the size,’ explains Chao-Yang. ‘Our fast-charging battery technology is precisely aimed at downsizing batteries for electric vehicles without letting customers feel range anxiety.’
Reduce, reuse, recycle
At the University of Leicester, chemist Andy Abbott’s team has developed an automated technology to recover elements from scrap left over from battery manufacturing. The technique uses ultrasound in the same way that a dentist uses it to remove plaque from teeth. ‘Plaque is a weakly held adhesive bonded to a very strong substrate. With a battery, you have an active material stuck with glue onto a current collector, which is either a copper or aluminium sheet,’ he says. The ultrasound blasts the materials apart.
The biggest problem for recycling batteries themselves is the polymers that glue battery cells together. Andy’s team is looking into new adhesives that would be straightforward to dissolve. One particular problem is the perfluorinated polymers used as binders inside batteries. ‘What we would really love is a water disposable adhesive. At the moment, these are available for the anode but not for the cathode,’ says Andy. His group is looking at new adhesives for the cathode and the anode, which would ‘transform the recycling industry’.
With so many aspects to focus on and the chance to make a difference, many students want to get into battery research. ‘It’s one of the few areas where you can take something that’s really fundamental and see it go all the way through to application in a reasonably short space of time,’ says Clare. ‘It’s quite cool.’
More resources
- Watch this video from a collection for 16–18 students, carry out the microscale experiments and use the supporting resources to help learners investigate and apply the principles of electrochemistry.
- Get your 16–18 learners practising calculations and predicting reactions using EƟ values with the Redox equilibria starter for 10.
- Meet Liz, a PhD researcher investigating new materials for lithium-ion batteries to improve their performance in technology.
- Provide context for life-cycle assessments when teaching your 14–16 learners with this worksheet on lithium-ion batteries and the issues surrounding their manufacture and disposal.
More resources
- Help learners investigate and apply the principles of electrochemistry by watching a practical video, carrying out microscale experiments and using the supporting resources: rsc.li/3VBsK2E
- Get your 16–18 learners practising calculations and predicting reactions using EƟ values with the Redox equilibria starter for 10: rsc.li/3F8FDdI
- Meet Liz, a PhD researcher investigating new materials for lithium-ion batteries to improve their performance in technology: rsc.li/3h8MgVk
- Provide context for life-cycle assessments when teaching your 14–16 learners with this worksheet on lithium-ion batteries and the issues surrounding their manufacture and disposal: rsc.li/3UHnGIK
Article by science writer Emma Davies. Resource by Sue Ali, head of science at Harrogate Ladies’ College
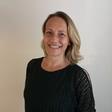
No comments yet