Discover the new biosensors, food testing systems and functional textiles that may soon change your life
The radiofrequency waves that surround us may be invisible, but researchers are looking at ways to harness their energy to power flexible biosensors that stick to skin and measure molecules in our sweat. Information from the sensors would not only help monitor an individual’s health but would also give valuable insights into the health of a given population.
These types of devices would not be possible without nanomaterials such as graphene and metal nanoparticles. Nanomaterials have a high surface area and magnetic, optical and mechanical properties that differ substantially from their bulk counterparts. Their properties can be tuned by controlling their size and shape and by adding different functional groups or coatings.
Many nanomaterial applications don’t make it beyond the research stage, with scale-up and commercialisation proving too tricky and expensive. Yet others are widely used and readily available. Silver nanoparticles are used in anti-microbial applications because of their capacity to damage bacterial cell walls. You will find them in anti-odour items such as sports shoes and socks. Meanwhile, textiles coated with different metal oxide nanoparticles can be used for UV-resistant clothing.
In your class
Nanoscience can feel like science fiction rather than science fact to learners. The applications presented in exam specifications are relatively limited and also long-established: nanoparticles were first used in sunscreens in 1980. Use this article to introduce learners to the most up-to-date applications of nanoscience. Follow up by using the accompanying structure strip resource to focus learners on the core learning objectives for 14–16 in the area of nanoscience.
Good old graphene
One of the most well-known nanomaterials is graphene. It was first isolated from graphite in 2004, to much fanfare, although it has failed to live up to the initial commercial hype.
Graphene is a two-dimensional material with exceptional mechanical, electrical and thermal properties, comprising a single layer of carbon atoms arranged in a hexagonal lattice structure. It is widely studied for its potential use in various fields such as electronics, energy storage and biomedicine.
Huanyu (Larry) Cheng, an engineer at Pennsylvania State University, uses graphene in his work to develop self-powered wearable biosensors, for monitoring substances such as glucose.
The researchers have tested a human motion-driven, self-powered stretchable sensor
Some consumers are interested in monitoring their glucose levels so they can tune their diets to prevent sugar spikes and crashes. For people with diabetes, being able to monitor glucose levels is an absolute necessity. Most sensors use small needles to access blood but being able to monitor glucose through sweat is highly desirable.
Larry is looking at ways to make the flexible biosensors power themselves. Options include harvesting the energy from radiofrequency waves, or using the body’s thermal energy or its kinetic motion.
Download this
Structure strip on the topic of nanomaterials, for age range 14–16
Use this writing prompt to prepare notes on the topic of nanomaterials and the nanoscale before answering an extended response question.
Resources include:
Download this
Structure strip on the topic of nanomaterials, for age range 14–16
Use this writing prompt to prepare notes on the topic of nanomaterials and the nanoscale before answering an extended response question.
Download the resources from the Education in Chemistry website: rsc.li/3s2hinh
Electrochemical sensors
Larry uses existing technology for harvesting energy but adapts it to create stretchy, creating spring-like serpentine structures that move with the skin. For example, his team has made stretchable microstrip antennae using a UV laser.
His team’s flexible and wearable devices are mainly based on laser-induced graphene (LIG), a porous, three-dimensional graphene foam, which would not be suitable for computing but is perfect for bendy sensors. The LIG provides an efficient flow of electrons and can host nanocrystals such as metals or metal oxides, creating conductive carbon electrodes for flexible devices. The researchers have tested a human motion-driven, self-powered stretchable sensor based on LIG foams with embedded gold and manganese oxide nanocrystals.
For glucose sensing, they plated nickel and gold onto LIG electrodes. In tests, they have recorded accurate glucose measurements from human sweat, which ‘opens up opportunities’ for wearable electronics. ‘We are developing this as a platform where we can create different material composites for different biomarkers,’ says Larry.
The researchers say their fabrics are ‘virtually non-combustible’ and predict next-generation flame-retardant textiles
Scale-up and commercialisation will be a challenge, but he feels that their choice of CO2 laser for making the LIG is a good strategy because it is commonly available. If the sensors can be made low cost and affordable, then Larry envisages being able to collect a large amount of data from wearers – for example from people in a certain region or in a hospital, using artificial intelligence to draw meaning from it.
With increasing interest in personal air quality monitoring and breath analysis, Larry’s team has also created sensors for nitrogen oxides (NOx), major atmospheric pollutants known to adversely affect human health. These sensors have an LIG electrode region sandwiched between a semi-permeable membrane and a soft substrate.
A team led by Wei Fan from Xi’an Polytechnic University in China is using graphene to create fire-resistant suits for firefighters with built-in sensors for toxic nitrous oxide gas, which forms during combustion processes. The woven fabric contains graphene and poly(p-phenylene benzobisoxazole) fibres. Electrodes for sensing gas are stable at temperatures over 520°C, they claim. The researchers say their fabrics are ‘virtually non-combustible’ and predict that they could lead to next-generation flame-retardant textiles for ‘smart firefighting’.
Contaminated food
Many groups are using nanomaterials in sensors to detect food that is contaminated or spoiled by bacteria. According to the World Health Organization, food poisoning affects 600 million people per year. Bacterial tests detect food poisoning bacteria during food manufacturing, but it generally takes more than 48 hours for the cultures to grow.
At Osaka Metropolitan University in Japan, scientists have developed a simple, rapid method to identify multiple food poisoning bacteria, including Escherichia coli and Staphylococcus aureus. The technique uses colour differences in scattered light from nanometre-scale organic metal nanohybrid structures designed to bind to the bacteria.
A team led by Hiroshi Shiigi has made organic metal nanohybrids comprising nanoparticles encapsulated in polymer particles, adding antibodies that bind specifically to E coli and S aureus. The light-scattering characteristics of the nanohybrids depend on the metals in the nanoparticles. For example, gold, silver and copper nanohybrids produce white, red and blue light, respectively. Using a dark field microscope, they can rapidly identify different bacteria based on the colour of the scattered light from the antibody-adapted nanohybrids.
At McMaster University in Ontario, Canada, researchers are working on DNA-based probes to detect bacteria in food using a handheld fluorescence reader or a colorimetric system. They have developed probes for Salmonella and E coli and are working on one for Listeria.
More resources
- Read about the sunlight-activated nanofilm putting an end to misty glasses and windows, and use the accompanying summary slide to add new context when teaching about nanomaterials at 14–16.
- Use the example of nanotechnology in socks to introduce ethics in science with this structured practice debate.
- Get your students to take part in the Nanoparticles in sunscreen challenge by making sunscreen and determining its SPF using UV light transmission.
- Inspire your students with games, activities, practicals and demonstrations on the topic nanotechnology.
More resources
- Read about the sunlight-activated nanofilm putting an end to misty glasses and windows, and use the accompanying summary slide to add new context when teaching nanomaterials at 14–16: rsc.li/46DMwjx
- Use the example of nanotechnology in socks to introduce ethics in science with this structured practice debate: rsc.li/45EyLjh
- Get your students to make sunscreen and determine its SPF by using UV light transmission: rsc.li/3M8pWaL
- Inspire your students with games, activities, practicals and demonstrations on the topic nanotechnology: rsc.li/45F8Nfq
‘We are not detecting the bacteria themselves, but what they secrete, which makes it easier for us because we don’t need the sensor to reach the actual bacteria,’ says engineer Tohid Didar. He works with biochemists who find nucleic acids for the probes. ‘So, if our target is Salmonella, we throw it in a bath of DNA and then we see which sequences attach to it.’ The researchers then amplify the DNA sequence and test it against other targets that they don’t want it to bind to, such as good bacteria.
They also test the sequence against fresh meat to make sure it doesn’t trigger a signal. ‘At the end of the process we discover a sequence – a probe – that we know has a high specificity towards, say, Salmonella,’ explains Tohid.
Depending on whether they want to use fluorimetric or colorimetric detection, the team attach fluorescence molecules or use coloured nanoparticles like gold. The nucleic acid probes cleave in the presence of a bacterial ribonuclease enzyme, which frees the nanoparticles. For the fluorescent systems, the probe is attached to a fluorescent molecule with a quencher attached. ‘When the target comes it removes that cap and the fluorescent signal increases,’ Tohid says.
Tohid’s team has developed a smart ‘Lab-in-a-package’ with sensors built into food packaging trays. ‘If you want to do an assay, you need reagents,’ says Tohid. ‘We included a tiny membrane inside the packaging that’s like a sponge that holds some of the reagents that are also food-friendly.’ Packaged foods are generally randomly tested for contamination but ‘you don’t know what is going on in each of the packages,’ he explains. ‘With this system, you will know what’s going on inside.’
Tohid is also working on a ‘multiplex’ system. ‘The goal is to put this in some sort of barcode format, so you scan for multiple pathogens at the same time,’ he says. The team has had commercial interest but scale-up is challenging, particularly with keeping costs low. For commercialisation, the product will also need to comply with regulations in different regions.
Other teams are working on different types of food contamination. In 2022, an international team led by Arben Merkoçi at the Autonomous University of Barcelona in Spain developed a sensor to detect if beef meat is contaminated with pork. Such diagnostic tools are especially important where beef must be free from pork residues for religious, cultural or allergy reasons.
The sensor contains a DNA probe specific for the pork mitochondrial genome, immobilised on carbon electrodes modified with graphene acid, which improves the charge transport properties. In trials, the sensor detected pork residues in under 45 minutes. The platform has the potential to immobilise other DNA sequences for a ‘multitude of targets’ and so could be used for other food systems or crops, they say.
Nanomaterial-based sensors are difficult to design but then relatively easy to tweak for different targets. The snag, as ever, is making systems that researchers can scale up and commercialise.
Article by Emma Davies, a science writer based in the UK. Structure strip resource by Kristy Turner, a school teacher fellow at The University of Manchester
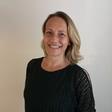
No comments yet