Solidify students' understanding of this fundamental law with these hands-on teaching ideas
As early as the 6th century BCE, Indian philosopher Kanada speculated that matter was made of tiny, indivisible particles and that although they may change in their arrangement, their fundamental nature would remain unchanged. However, it wasn’t until more than 2000 years later, in 1789, that Antoine Lavoisier proved this theory when he discovered the law of conservation of mass. The law demonstrates that no atoms are lost or made during a chemical reaction.
Lavoisier’s theory was transformative, because the understanding of conservation of mass is central to our understanding of reactions and therefore chemistry. The concept links the macroscopic world of measurable masses to the microscopic world of atoms and molecules.
Suggestions for teaching
Learners often have everyday questions that link to this principle, such as where does sugar go when we dissolve it in tea, or why are the ashes from a fire lighter than the wood and coal that burn. In both of these examples, students may be confused about where the mass goes.
Use questions like these to activate learners’ thinking and prior knowledge, before exploring conservation of mass more deeply and the differences between open and closed systems.
What students need to know
All 11–14 students should be familiar with key terms, such as atoms, molecules and compounds, and will know that a chemical reaction involves a rearrangement of atoms. They will also have learned about states of matter and the properties of solids, liquids and gases.
At 14–16, learners need to know:
- Atoms are neither created nor destroyed during a chemical reaction.
- In closed systems, in which no matter enters or escapes, the mass remains constant.
- In open systems, gases produced or consumed can give the false impression that mass has been lost or gained.
- The law of conservation of mass can be shown on a symbolic level in a balanced symbol equation in which the number of atoms of each element is the same on both sides of the reaction.
Common misconceptions
Among the misconceptions learners bring to this topic is the idea that gases are lighter than liquids and solids. Gases are less dense than liquids, which are in turn less dense than solids. Learners can confuse a reduction in density of a substance with a reduction in mass, whereas this is not the case because the number of particles remains the same.
Easily demonstrate this to students easily by allowing some ice to melt in a beaker and measuring the mass before and after to show that the mass doesn’t change.
Another common misconception is that chemical reactions occur until all the reactants are used up. This error occurs most often for reactions that finish without any solid visible, for example the reaction between an acid and an alkali or magnesium reacting with acid. Help your learners to understand that one reactant will often be in excess, and that the limiting reactant dictates when the reaction finishes.
To address this, you can ask students ‘why has this reaction finished?’, to prompt them to think about which reactant has been limiting and which was in excess. When calculating reacting masses, divide the number of moles of a substance by the stoichiometric number. The reactant with the smallest number is the limiting one.
Checking for understanding
You can use Johnstone’s triangle when teaching conservation of mass to bridge the gap between reality and what can feel like an abstract concept to learners at this stage of their education.
Macroscopic level
The macroscopic element of conservation of mass can be introduced by using reactions in which neither the reactants nor the products are gases. Precipitation reactions are perfect for this, such as the reaction between potassium iodide and lead(II) nitrate(V).
Demonstrations that cause a change in mass, such as the oxidation of magnesium, and various reactions in which a gas is produced, occur in open systems. These demonstrations provide a stimulus for learners to consider the difference between these reactions and the precipitation reaction and can lead to valuable discussions.
To illustrate the difference between an open and a closed system, you can carry out an experiment with vinegar and baking soda in an open conical flask on a mass balance, which will lead to a decrease in mass as carbon dioxide escapes. You can then show the differences between an open and a closed system by repeating the demonstration with a balloon over the neck of the conical flask to close the system.
Finally, to deepen understanding, you can introduce simple reacting mass calculations. By using the experiment without the balloon, ask students how they can determine how much CO₂ was produced. The answer lies in calculating the difference in mass between the starting reactants and the remaining products.
Submicroscopic level
The second element of Johnstone’s triangle is submicroscopic. Use modelling kits (or even playdough and cocktail sticks) to allow students to demonstrate what they have learned about the conservation of mass from experiments and the surrounding discussions. Give them opportunities to get hands-on experience and observe the rearrangement of atoms, noting that there are the same amount of atoms before and after rearrangement.
Symbolic level
The final stage is the symbolic level of Johnstone’s triangle. Students learn how to balance equations, with a firm understanding of why this is necessary. This stage connects the theoretical and practical aspects they’ve encountered with a more abstract, mathematical approach – balancing chemical equations. This stage also consolidates the principle that the number of atoms for each element remains the same before and after a reaction.
The journey from hands-on demonstrations to balancing symbol equations fosters both confidence and curiosity, and prepares students for post-16 challenges, such as calculating reacting masses, using molar ratios and identifying limiting reactants. These concepts are also important in industrial processes in which maximising both percentage yield and atom economy is crucial for green chemistry and to ensure the sustainability of the chemical industry.
More resources
- Use these teacher-tested ideas to introduce the topic of conservation of mass to 11–14 learners
- Show learners the fundamental law in action, in open and closed systems, with the Conservation of mass practical video
- Help students visualise the different components of chemical reactions with downloadable slides and worksheets for the classroom
More resources
- Recap prior knowledge introduced at 11–14 with teacher-tested ideas for teaching conservation of mass and help students visualise the different components of chemical reactions with this article and accompanying classroom activity.
- Show learners the fundamental law in action, in open and closed systems, with the Conservation of mass practical video.
- Reinforce your learners' understanding of quantitative chemistry with review my learning worksheets
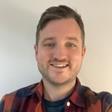
7 readers' comments