I love operating in the space between the kinetic and the thermodynamic. It’s here where the seemingly simple becomes complex, and it’s a reminder of the precious interplay of time and energy that allows life to eke out an existence. It’s in this space that we can answer questions like; should alkali metals explode in water? Why don’t diamonds turn into graphite? How do clouds form? Why does food explode in a microwave? And we can explain oscillating reactions. I have a fascinating fizzy fluctuation for you to explore oscillating reactions with your learners.
Get started
Watch a demonstration of this experiment and download the technician notes from the Education in Chemistry website: rsc.li/3MNpNJh
I love operating in the space between the kinetic and the thermodynamic. It’s here where the seemingly simple becomes complex, and it’s a reminder of the precious interplay of time and energy that allows life to eke out an existence. It’s in this space that we can answer questions like; should alkali metals explode in water? Why don’t diamonds turn into graphite? How do clouds form? Why does food explode in a microwave? And we can explain oscillating reactions. I have a fascinating fizzy fluctuation for you to explore oscillating reactions with your learners.
Kit
- 2.6 g ammonium sulfate(VI)
- 10 cm3 0.2 M sulfuric(VI) acid
- 2.7 g sodium nitrate(III)
- 10 cm3 deionised water
- Two 100 cm3 beakers
- Clean borosilicate boiling tube (see Top tips)
- Boiling tube rack
Preparation
Separately prepare the two solutions in 100 cm3 beakers. Make the acidified 2 M ammonium sulfate(VI) solution by dissolving 2.6 g solid ammonium sulfate(VI) in 10 cm3 of 0.2 M sulfuric(VI) acid. Dissolve 2.7 g solid sodium nitrate(III) in 10 cm3 of deionised water to make the ≈3.9 M sodium nitrate(III) solution. Stir each solution thoroughly until completely dissolved. For the supersaturation effect to work best, there should be no pieces of solid present.
Safety and disposal notes
- Work in a fume cupboard.
- Wear eye protection.
- Avoid skin contact.
- Sodium nitrite is oxidising, toxic and toxic to aquatic life.
- Do not mix the solids directly.
- Take care, the boiling tube will be hot.
In front of the class
Wear eye protection and work in a fume cupboard, as oxides of nitrogen will evolve. Pour the contents of one beaker into the other, mix thoroughly and decant immediately into a boiling tube. Brown fumes of nitrogen dioxide (NO2) will be produced and the reaction will fizz, which will pulse every ≈10 seconds for 5–10 minutes.
Teaching goal
The initial brown gas evolved forms by disproportionation of nitric(III) acid (nitrous acid) at low pH to release nitrogen monoxide (NO) and NO2. As the pH increases, this process slows, and the show begins. The nitrate(III) ions react in solution with ammonium ions to form nitrogen (see equation below). Initially, this gas dissolves in the solvent but the solubility of nitrogen in water is very low – 0.0019 g/100 g water at 1 atm, so ≈100 times less soluble than carbon dioxide.
NH4+ (aq) + NO2- (aq) → N2 (g) + 2H2O (l)
The nitrogen could diffuse to the surface and escape, but typical small molecule diffusion speeds in water are slow (≈millimetres per minute) and the reaction produces gas quickly. So, for the gas to escape, a bubble needs to form. Without seeding (see Hot ice), a bubble needs to nucleate from random fluctuations in the solution.
Essentially, although gas evolution is predicted from a thermodynamic perspective, there is a kinetic barrier. So, the dissolved gas supersaturates to concentrations 80 times the predicted solubility at 1 atm. At this point, there is a good chance that a randomly appearing nucleation site will be filled with gas quickly enough to reach the critical size needed to survive and grow. The concentration-dependence of this threshold has been shown to be so strong that, at the threshold of supersaturation, a 0.1% change in the concentration of the dissolved nitrogen will lead to a 1015-fold increase in the rate of bubble formation. Unusually for a kinetic effect here, the temperature has less of an impact than concentration. This is helpful because the reaction is strongly exothermic. Although frothing peaks between 2 and 4 minutes, the temperature rise does not slow until >4 minutes, peaking at ≈70°C, after 9 minutes.
As the bubbles rapidly nucleate, the surrounding supersaturated liquid now has an increased surface area the dissolved gas can evaporate across. Bubbles continue to grow until they can escape to the surface (although they may stop increasing in size if the concentration of the dissolved gases drops sufficiently). With such an explosion of bubbles, the dissolved gas is rapidly exhausted and the foaming decreases. Yet, nitrogen continues to be produced, which leads to the next supersaturation event ≈10 seconds later.
This phenomenon explains frothing when you pour a fizzy drink, and the different tones and volumes associated with a boiling kettle.
Teaching goal
The initial brown gas evolved forms by disproportionation of nitric(III) acid (nitrous acid) at low pH to release nitrogen monoxide (NO) and NO2. As the pH increases, this process slows, and the show begins.The nitrate(III) ions react in solution with ammonium ions to form nitrogen. Initially, this gas is dissolved in the solvent but the solubility of nitrogen in water is very low – 0.0019 g/100 g water at 1 atm, so ≈100 times less soluble than carbon dioxide.
The nitrogen could diffuse to the surface and escape, but typical small molecule diffusion speeds in water are slow (≈millimetres per minute) and the reaction produces gas quickly. So, for the gas to escape, a bubble needs to form. Without seeding, a bubble needs to nucleate from random fluctuations in the solution.
Essentially, although gas evolution is predicted from a thermodynamic perspective, there is a kinetic barrier. So, the dissolved gas supersaturates to concentrations 80 times the predicted solubility at 1 atm. At this point, there is a good chance that a randomly appearing nucleation site will be filled with gas quickly enough to reach the critical size needed to survive and grow. The concentration-dependence of this threshold has been shown to be so strong that, at the threshold of supersaturation, a 0.1% change in the concentration of the dissolved nitrogen will lead to a 1015-fold increase in the rate of bubble formation. Unusually for a kinetic effect here, the temperature has less of an impact than concentration. This is helpful because the reaction is strongly exothermic. Although frothing peaks between 2–4 minutes, the temperature rise does not slow until >4 minutes, peaking at ≈70°C after 9 minutes.
As the bubbles rapidly nucleate, the surrounding supersaturated liquid now has an increased surface area the dissolved gas can evaporate across. Bubbles continue to grow until they can escape to the surface (although they may stop increasing in size if the concentration of the dissolved gases drops sufficiently). With such an explosion of bubbles, the dissolved gas is rapidly exhausted and the foaming decreases. Yet, nitrogen continues to be produced, which leads to the next supersaturation event ≈10 seconds later.
This phenomenon explains frothing when you pour a fizzy drink and the different tones and volumes associated with a boiling kettle.
Top tips
- Ensure you use sodium nitrate(III) (sodium nitrite), not sodium nitrate(V).
- Tall vessels (like boiling tubes) maximise the frothing effect.
- Try up-lighting to visualise the bubbles better.
- Seeding disrupts the supersaturation effect. The pulsing effect stops with the addition of anti-bumping granules. For this reaction to work, a high degree of supersaturation is crucial, so the boiling tube must be clean. I used a new tube, but a clean, undamaged tube will work too.
- Direct students to focus on the bulk solution. A visualiser can help. Students may notice some bubbles clinging to the side of the tube and ask why these don’t relieve the supersaturation: the answer lies in the short distance (only ≈0.1 mm) a dissolved gas molecule could diffuse in the time between the 10-second pulses, which leaves these bubbles essentially divorced from the solution in the centre of the tube.
More resources
If you’re looking for more demonstrations related to the topics in this article, see these resources:
- Demonstrate the gallium beating heart and the Briggs-Rauscher reaction when teaching oscillating reactions.
- Use a practical on the reactions of the alkali metals to show your students the interplay between kinetic and thermodynamic effects.
- Create hot ice to show your students the effects of supersaturation.
Downloads
Let's get fizzical technician notes
Handout | PDF, Size 0.15 mbLet's get fizzical technician notes
Editable handout | Word, Size 0.45 mb
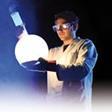
No comments yet