Secure 14–16 student understanding of this topic with these activities, ideas and misconception busters
The concept that chemical reactions are reversible and exist at an equilibrium point is often challenging for students to understand. Before 14–16 learning, students are often taught that chemical changes are irreversible. Reversible reactions and equilibrium underlie many topics of global relevance, including the economics of the Haber and Contact processes, climate change and ocean acidification. Oxygen binding to haemoglobin and the maintenance of the pH of our blood are other great contexts to help students understand the relevance of reversibility and equilibrium.
What students need to know
- Chemical reactions involve the rearrangement of atoms and the breaking and forming of bonds.
- Changes of state in molecular substances involve changes in the bonding between the molecules, but not within them.
- Chemical reactions can be exothermic or endothermic, and temperature affects how reactions progress.
- Concentration is a measure of the amount of substance in a set volume.
- Reactions are observed macroscopically, but changes occur sub-microscopically.
Threshold concepts
When introducing equilibrium to students, start with familiar examples. Ask questions such as: what happens when we leave an open glass of water in a room? Most students will be able to tell you that, over time, the level of the water in the glass will decrease as the water evaporates into the atmosphere. We can then ask: what happens when we have the same glass, but with a lid on it? Students may suggest that the water level will remain constant (a macroscopic observation) because the rate of evaporation and condensation are equal (a sub-microscopic explanation). Writing out the equation of these reactions explicitly (symbolically) can help develop their understanding of the different ways we view chemical change:
- Evaporation: H2O(l) → H2O(g)
- Condensation: H2O(g) → H2O(l)
- Overall: H2O(l) ⇌ H2O(g)
Once students understand this, consider other reactions that you can reverse by changing the conditions, such as the decomposition of ammonium chloride. In this reaction, ammonium chloride (a solid) thermally decomposes to give ammonia and hydrogen chloride (gases) when heated. However, when the gases are allowed to cool, the reverse reaction occurs. Another good example of a common reversible reaction is the (de)hydration of copper sulfate.
You can look at cobalt complexes to help students develop an understanding of the position of equilibrium: [Co(H2O)6]2+ + 4Cl– ⇌ [CoCl4]2– + 6H2O. In a solution with a low concentration of chloride, cobalt forms a pink complex with water molecules. As the chloride concentration increases, cobalt forms a blue complex with chloride ions. The dative covalent bonding involved in these complexes is not explained by the bonding model usually taught at ages 14–16. However, it provides a useful hint at the more complex bonding models introduced at post-16.
Starting from a mixture of the two complexes, which is purple, explore the factors that affect the equilibrium position, in particular concentration and temperature. The addition of chloride causes the equilibrium position to shift to the right, so the solution turns from purple to blue. In chemistry for 14–16 year olds, we account for this change using Le Chatelier’s principle, which states that the equilibrium will shift to oppose the change. The shift in equilibrium position (due to greater relative concentration of [CoCl4]2–), which is a sub-microscopic change, is observed macroscopically as a colour change (more blue compound, less pink compound). You can explicitly refer to Johnstone’s triangle to help students understand the macroscopic, sub-microscopic and symbolic representations discussed.
Le Chatelier’s principle can account for the effect of changing the temperature of reactions. When reactions are heated, the equilibrium position shifts. The additional energy is absorbed by the system, which favours the endothermic reaction. This experiment also shows that an equilibrium can be approached from either direction. Move a tube of the mixture between hot and cold water baths and watch as the colour changes back and forth.
We need to be careful with Le Chatelier’s principle. It’s a good descriptive tool, but can lead students to a simplistic mental model of equilibrium. The mathematical description given at post-16 level challenges this – it shows that the rate of both forward and reverse reactions change when conditions change, and a new equilibrium is only reached once these two rates are again equal.
We need to be careful with Le Chatelier’s principle. It’s a good descriptive tool, but can lead students to a simplistic mental model of equilibrium. The mathematical description given at post-16 level (rsc.li/3tq2Q8I) challenges this – it shows that the rate of both forward and reverse reactions change when conditions change, and a new equilibrium is only reached once these two rates are again equal.
Clearing up misconceptions
Students often think that because there is no observable macroscopic change at equilibrium, the rate of the forward and reverse reactions is zero, or that there are no changes occurring in the reaction container. Diagnostic questions can help uncover students’ views. Thought experiments, like considering what you observe macroscopically when you walk up an escalator at the same rate as it is moving downwards, can help students recognise this misconception. Simulations and models can also help learners understand what’s happening sub-microscopically.
Students may not have considered the difference between open, closed and isolated systems. Use simple diagrams to summarise the different types of system.
Learners often think that the concentration of reactants and products is equal at equilibrium, and so confuse concentration and rate. Get them to sketch concentration (of reactants and products) versus time, and rate (of the forward and reverse reactions) versus time graphs, to show what happens when reaching equilibrium. Emphasise that, at equilibrium, concentrations are steady (but not necessarily equal), and rates are steady (and always equal).
Students may view the forward and reverse reactions as separate from each other, assuming that one can be changed without the other. The mathematical approach used at post-16 can support here. At 14–16, you may want to explicitly discuss that a reaction is one system, and that a change in any component will affect both the forward and reverse reactions.
Checking for understanding
- The concepts of reversible chemical reactions and equilibriums will likely conflict with what students learned at 11–14 in chemistry and physics.
- Use familiar scenarios to help students access new ideas and understand their relevance.
- Explicitly discuss how, sub-microscopically, both the forward and reverse reactions are occurring at the same rate and that, macroscopically, we see no change.
- Use practical demonstrations which give students the opportunity to observe these changes. Demonstrate and clearly explain how these systems respond to changes.
- Use graph sketching and diagnostic questions to assess understanding at the sub-microscopic and macroscopic levels, and of symbolic representations.
Jo Haywood teaches at secondary school and undergraduate level as well as leading chemistry on the University of Cambridge PGCE course
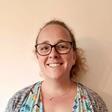
1 Reader's comment