Dietitians need to understand the chemistry behind how the body metabolises and uses different nutrients, as well as the mechanisms of common clinical conditions
Complex health conditions such as diabetes are on the rise. dietitians are perfectly placed to help provide solutions by translating nutritional science into practical dietary advice. They also play a role in helping to prevent certain diseases through diet. dietitians work for the NHS and private clinics, as well as for the food industry and sports bodies. They also help governments and local communities to develop food and health policy.
To practise as a dietitian in the UK, you must be registered with the Health and Care Professions Council. This requires an approved qualification in dietetics, generally a BSc degree or a postgraduate qualification. Most university dietetics BSc courses require applicants to have A-levels, or the national equivalent, in both biology and chemistry. It’s easy to see why a scientific background is essential. dietitians need to understand exactly how the body metabolises and uses different nutrients, as well as the mechanisms of common clinical conditions, such as type II diabetes.
Dietetics courses also delve into a raft of chemistry topics taught at 16–18, including bonding, acids and bases, pH and buffers, reaction kinetics and isomers.
In your class
Share this article and accompanying worksheet with your students to show them how their current studies are relevant to real-world careers. You could also refer to the specific ways that topics you are covering in class link to a future career as a dietitian as they come up.
Food groups
University dietetics courses cover the structures and functions of the three main types of nutrients essential for a healthy diet: proteins, carbohydrates and fats.
The large (macro)molecules are all made from smaller units linked together, from fatty acids in fats to amino acids in proteins. When foods are digested, glucose, fatty acids, small peptides and amino acids are the major absorbed end products.
Lipids, which include waxes and fats, are made from fatty acids attached to an alcohol called glycerol. The body breaks lipids down into fatty acids, needed for energy and to make cell linings and hormones.
Carbohydrates provide energy. They come in the form of simple sugars, such as glucose, double sugars (disaccharides), and complex carbohydrates, such as cellulose and starch, which consist of long chains of sugars. The body uses a series of enzymes to convert glucose and other simple sugars into pyruvate, a process that releases energy.
Type II diabetes is characterised by high levels of sugar in the blood. The hormone insulin instructs cells to take up glucose so it can be used to generate energy. When levels in the blood rise, for example after a meal, the pancreas produces more insulin. But in type II diabetes, the body’s cells resist insulin’s instructions and so glucose builds up in the blood. Over time, the pancreas becomes worn out and cannot keep up with the demand for ever-increasing insulin levels. dietitians can help patients to manage their blood sugar levels through diet.
Download this
This worksheet (available as MS Word or pdf) poses questions about carbohydrates, proteins, fats and enzymes – the building blocks of the knowledge required by dietitians to conduct their work – asking students to recall their own knowledge along with information from the article. They will see which parts of their learning are relevant to studying dietetics at degree level and ultimately to becoming a dietitian.
Protein-packed power
Amino acids, proteins and DNA feature in syllabuses at 16–18 as the ‘molecules of life’. Our bodies contain thousands of different proteins, each with a specific function. They provide structural components for cells and tissues, as well as enzymes and hormones.
A typical protein comprises 300 or more amino acids, linked by peptide bonds, with the specific number and sequence unique to each protein. Hydrolysing proteins sets the amino acids free. After digestion, amino acids are absorbed into the bloodstream and delivered to cells where they can be reassembled into new proteins. The amino acids in proteins can be arranged in many different ways, with the number and sequence determining the final shape.
The body continually repairs and replaces proteins and so requires a regular supply of amino acids. Although some can be reused when proteins break down, we need dietary protein to meet demand.
Enzyme action
Enzymes are biological catalysts that speed chemical reactions in the body. They are mainly globular proteins, roughly shaped into a ball, and typically increase the rate of a reaction by at least one million times. They attract molecules to an active site, effectively a crack or hollow in the enzyme ball. The active sites lower the activation energy for metabolic reactions to take place.
Each enzyme is highly specific, designed to fit a particular substrate. Molecules of just the right chemistry can fit into active sites, much like a key sliding into a lock.
Different digestive enzymes are designed to latch onto carbohydrates, proteins or fats. For example, an enzyme in saliva called amylase breaks down starch to form sugars such as glucose. Keep a morsel of white bread in your mouth for long enough and it will soon start to taste sweet, as the enzyme breaks down complex carbohydrates into simple sugars. Meanwhile, proteases digest proteins, while lipases break apart fats.
A catalase enzyme helps to protect the body from oxidative damage by neutralising hydrogen peroxide, a by-product of respiration, turning it into water and oxygen. In the lab, pupils may learn how catalysts such as manganese (IV) oxide can decompose hydrogen peroxide. Catalase does the same job, but at a rate that’s off the scale compared with inorganic catalysts.
Each enzyme has an optimal pH and so changing pH can affect the rate of enzyme-controlled reactions. The digestive enzymes trypsin and pepsin are good examples. They both break proteins into smaller bits or constituent amino acids, but pepsin works in the highly acidic conditions in the stomach while trypsin resides in the small intestine, with an optimal pH of about 8. Understanding why pH matters brings in ionic and hydrogen bonding, both key topics at 16–18. At very high or low pH, ionic bonds within an enzyme can be disrupted and it can lose its shape, preventing it from locking effectively to substrates.
Students are taught that amino acids themselves have interesting chemistry, with both acidic and basic properties, thanks to their amine and carboxyl groups. They can act as buffers, helping to stabilise pH. In strongly acidic solutions they behave as bases, with the carboxyl ion gaining a hydrogen ion while in alkaline conditions they behave as acids, losing hydrogen ions.
Although such knowledge may not be used by dietitians in their daily work, it really helps to understand what is going on at a molecular level before zooming out to provide practical dietary advice.
Find out more
You can download the Royal Society of Chemistry’s At School booklet (as a pdf) to show students the relevance of studying chemistry to future careers.
Explore the role of a scientist, Taryn, working in food and pharmaceuticals and find out what inspired them to work in chemical science.
Downloads
Chemistry of dietetics worksheet
Editable handout | Word, Size 85.13 kbChemistry of dietetics worksheet
Handout | PDF, Size 37.24 kbChemistry of dietetics - article text
Article | PDF, Size 38.29 kb
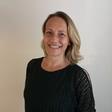
Why you need chemistry to study … at university
- 1
- 2
- 3
- 4
- 5
Currently reading
Dietetics at university
- 6
No comments yet